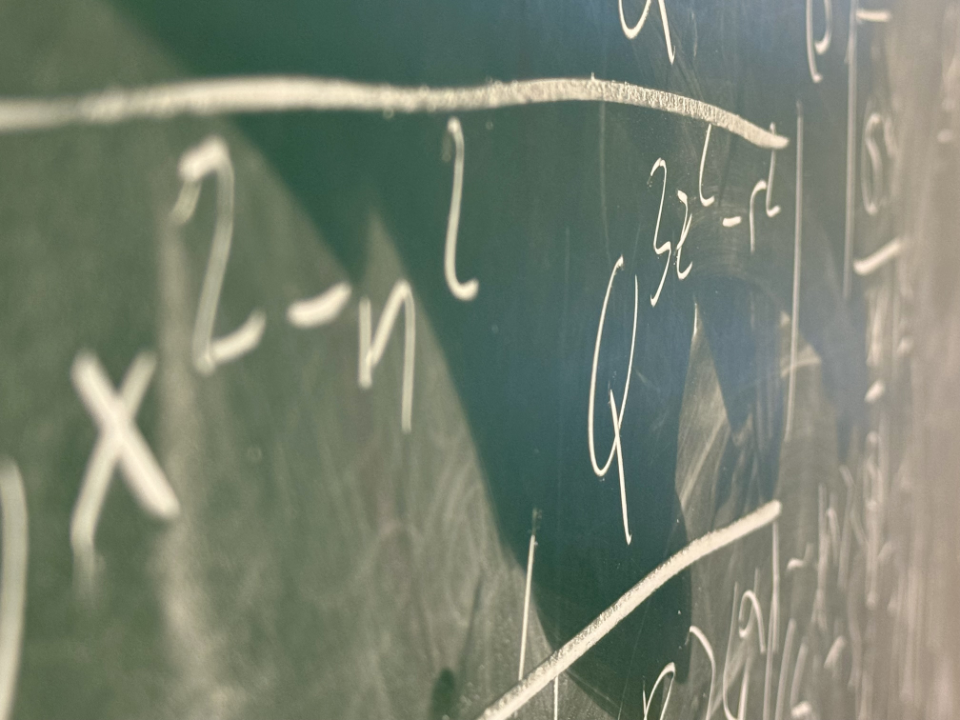
We investigate how interactions between quantum-mechanical degrees of freedom can give rise to novel emergent phenomena and unconventional phases of matter, and what signatures can be used to identify those in realistic experiments.
A particular motivation for our work is "quantum matter on demand", i.e. platforms that are specifically engineered and controlled (e.g. by driving with light) to exhibit states that are intrinsically reliant on quantum effects.
Frustrated magnetism and quantum spin liquids
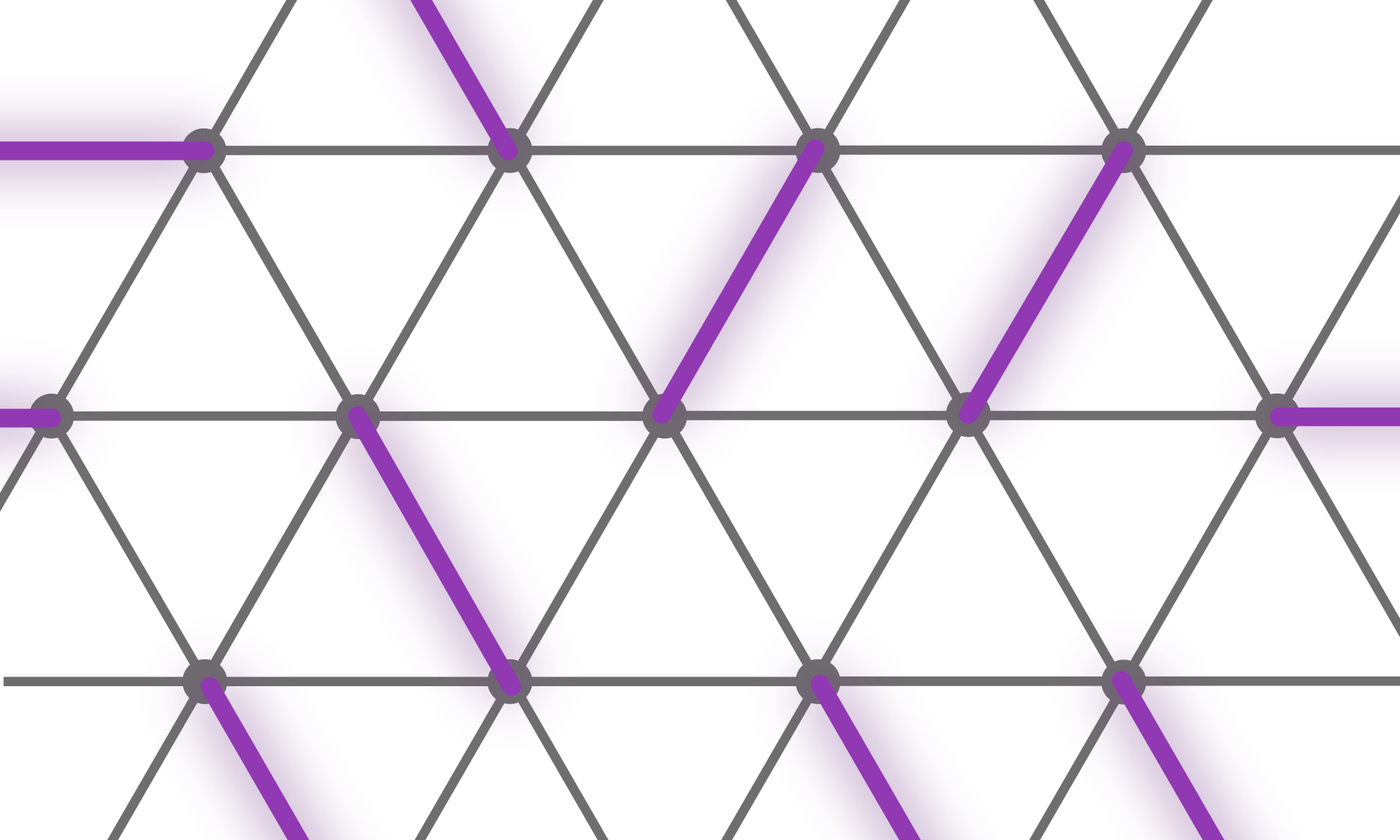
Quantum spin liquids are a paradigmatic examples for "quantum" phases of matter, featuring long-ranged entanglement patterns and fractionalized quasiparticles that carry quantum numbers that are different from any fundamental microscopic degrees of freedom. While there there has been significant progress in theoretically understanding these phases and identification of candidate materials, an unambigous experimental realization is still lacking.
We are especially interested in the robustness of these states upon coupling to other degrees of freedoms (such as phonons or itinerant electrons) and novel "descendant" phases that may emerge out of such coupling, and identifying new experimental signatures.
Moiré heterostructures and van der Waals materials
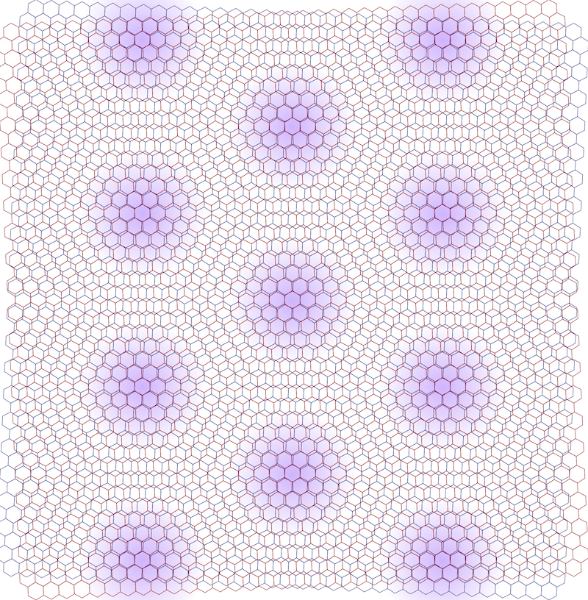
Moiré heterostructures are formed when atomically thin materials are stacked on top of each other with a small relative twist angle, or due to a small lattice constant mismatch in the two layers. The resulting "moiré lattice" can have lattice constants that are orders of magnitude larger than the individual atomic lattices. In such experimentally highly tuneable moiré heterostructures, a wealth of strongly-correlated states and phenomena, including Mott-Hubbard insulators, generalized Wigner crystals, superconductivity as well as Integer and Fractional Quantum Anomalous Hall states have been discovered.
We have been interested in moiré heterostructures of transition metal dichalcogenides (TMD) as a new platform for quantum magnetism. Current interests include heterostructures of intrinsically correlated layers as well as non-equilibrium phenomena in moiré TMD systems.
Optically driven quantum matter
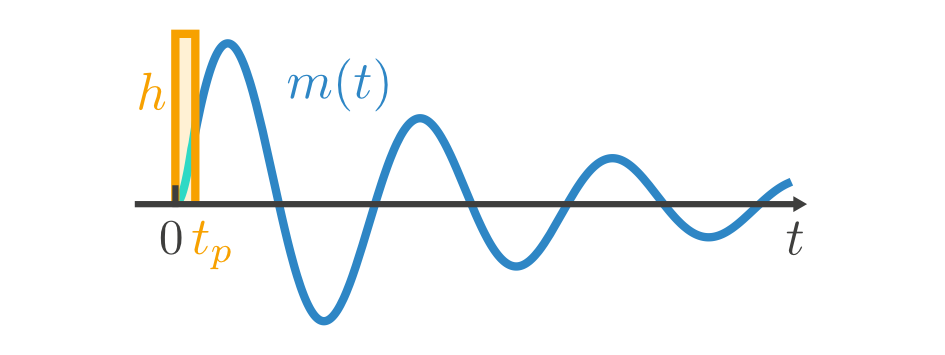
Ultrafast optical pump-probe spectroscopy is a powerful technique to study the dynamics of collective excitations, for example amplitude modes in superconductors or magnons in antiferromagnets. As an optical method, it is notably also applicable to 2D materials and heterostructures, in contrast to e.g. Neutron scattering methods which requires large sample volumes.
Going beyond using light to probe collective dynamics, theory and very recent experiments also suggest that irradiating quantum matter with light is an effective pathway for inducing novel interactions in a targeted manner and thereby stabilizing new desired states. An open question of interest to us is connecting theoretical model with realistic experimental settings.